- Climate
- Energy
In most cases, electrifying cars reduces their emissions
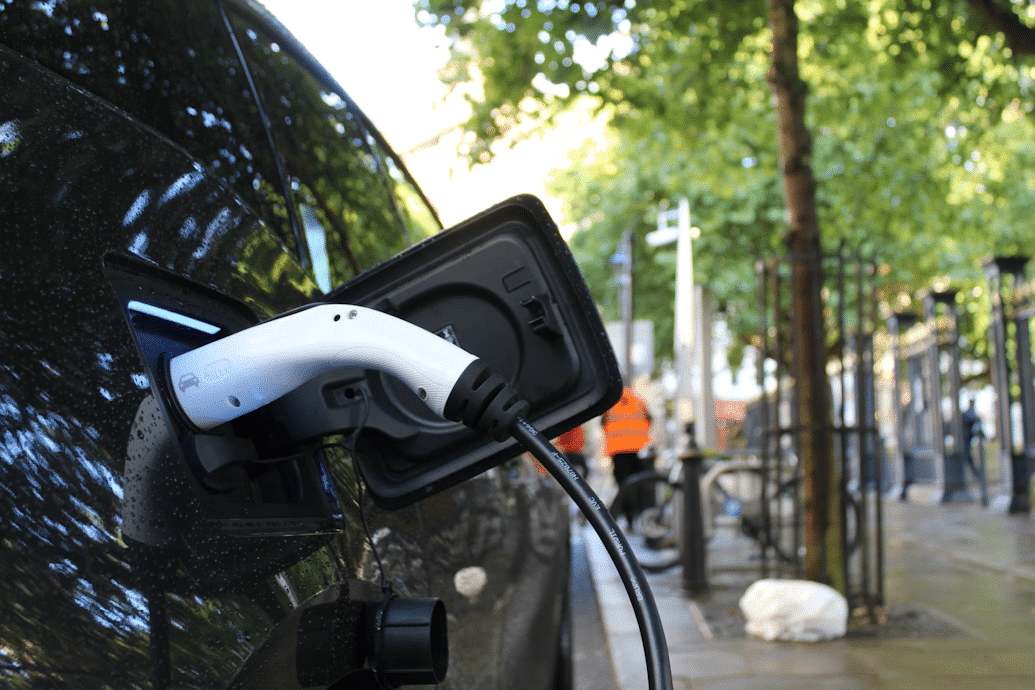
Today, road transport is responsible for 15% of the world’s total CO2 emissions. If we replace fossil-fuel-powered combustion vehicles with electric vehicles, we can reduce those emissions. This is one of the biggest reasons that many governments plan to end the sale of new fossil-fuel-powered vehicles by the 2040s. If current trends continue, electric vehicles will replace most of them on the market: nearly one in five new vehicles sold around the world in 2023 was an electric vehicle, up from around one in seven sold in 20221.
However, electric vehicle critics insist that the vehicles are not as “green” as they first seem. Critics often cite the lithium needed to craft the vehicles’ batteries or the fossil fuels that help generate their electricity, claiming that these things make them emit more greenhouse gas than comparable fossil-fuel vehicles. For example, see these past claims that Science Feedback has reviewed. Claims like these often cherry-pick data or ignore context, potentially misleading readers into perceiving electric vehicles as worse for the environment than they are in reality.
To properly assess a vehicle’s greenhouse gas footprint, we have to look at its emissions across its entire lifetime, from raw materials to recycling. We also have to compare an electric vehicle to its equivalent combustion vehicle: in other words, the car it would replace. Because burning fossil fuels in the engine is the largest source of a combustion vehicle’s emissions, electrifying that vehicle cuts out those emissions1. An electric vehicle may be more emissions-intensive to build than a combustion vehicle, and an electric vehicle may use fossil-fuel-fired electricity, but those differences are usually not large enough to make up for the lack of an in-car fuel tank2. Indeed, in most cases, an electric vehicle is responsible for fewer emissions over the course of its life than its combustion-powered counterpart3-5, as we explain in this article.
Main Takeaways:
- To properly compare vehicles’ emissions, we have to look at emissions across each vehicle’s entire lifecycle, from raw materials to recycling. On average, an electric vehicle emits less greenhouse gas over its lifetime than a comparable internal-combustion-powered vehicle.
- Batteries are greenhouse-gas-intensive to manufacture, and an electric vehicle’s production-related emissions are higher than those of an equivalent fossil-fuel-powered vehicle. But an electric vehicle makes up for those emissions as it operates, since it does not have an internal combustion engine producing emissions.
- In most parts of the world today, an electric vehicle is responsible for less greenhouse gas emissions than a comparable combustion vehicle. This is true even in places where electricity is primarily generated via fossil fuels.
- Electric vehicle emissions are poised to decrease in coming years, as manufacturing them becomes more efficient and as the electricity that powers them becomes less fossil-fuel-dependent.
Lifecycle analyses show that ELECTRIC VEHICLES have lower emissions on average
A vehicle isn’t just responsible for the emissions from its tailpipe. It’s also responsible for the emissions created from producing its raw materials, from turning those materials into a road-ready vehicle, from producing the electricity or the fuel that powers the drive, and from disposing of the vehicle at the end of its life. A life-cycle analysis aims to account for all of these stages.
When we look over the entire course of a car’s lifetime, most studies agree that replacing a combustion vehicle (ICEV) with an electric vehicle (EV) will reduce emissions. Rakesh Iyer and Jarod Kelly from Argonne National Laboratory provided the following comment to Science Feedback:
From the perspective of national and global decarbonization, there is clear scientific consensus that replacing internal combustion engine vehicles with electric vehicles reduces carbon emissions from a life-cycle perspective.
To better understand this, we can divide a vehicle’s emissions into two categories: those accrued while manufacturing the vehicle and those accrued while the vehicle is operating.
No matter what makes a vehicle go, it will accrue some emissions during its production: the costs of extracting raw materials and turning them into a functioning car. Here, an EV does have the additional emissions expense of sourcing and manufacturing batteries. In fact, data from the International Energy Agency (IEA) suggests that an EV’s production emissions are, on average, more than twice those of an equivalent ICEV1.
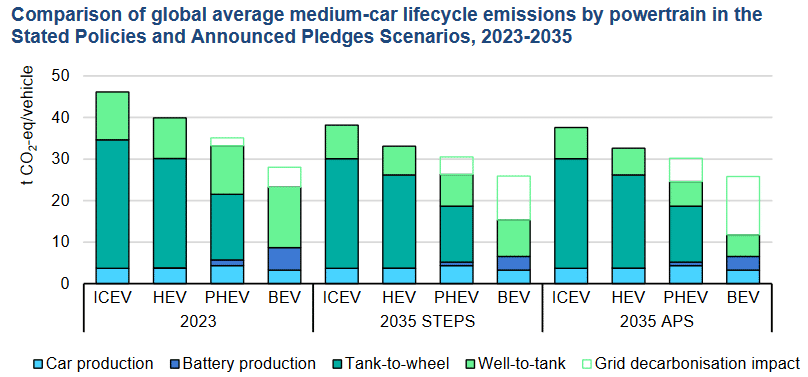
Still, most of a vehicle’s emissions occur as it operates. We can further split operating emissions in two types: those associated with bringing energy to the vehicle (sometimes called “well-to-tank” emissions; in the context of an ICEV, these are associated with turning crude oil into gasoline or diesel and bringing it to the car) and those created directly while operating the vehicle (sometimes called “tank-to-wheel” emissions).
An EV does accrue well-to-tank emissions: those associated with generating the electricity that charges it. But an EV, which isn’t burning fuel in the engine, does not generate tank-to-wheel emissions. This reduction is important, since tank-to-wheel emissions typically make up the largest part of an ICEV’s emissions (as shown in Figure 1). It is here that an EV tends to more than make up for its higher production emissions.
Consequently, we usually see that an EV is responsible for fewer total emissions across its lifecycle than an equivalent ICEV. According to IEA data, an EV’s lifecycle emissions in 2023 are 50% lower on average than those of an equivalent ICEV across the entirety of their respective life cycles. In fact, the IEA estimates that, by 2035, electrifying the vehicle fleet will avert 1.76 billion tonnes of operational greenhouse gas emissions under a scenario corresponding with current policies – more than the annual emissions of Brazil today1.
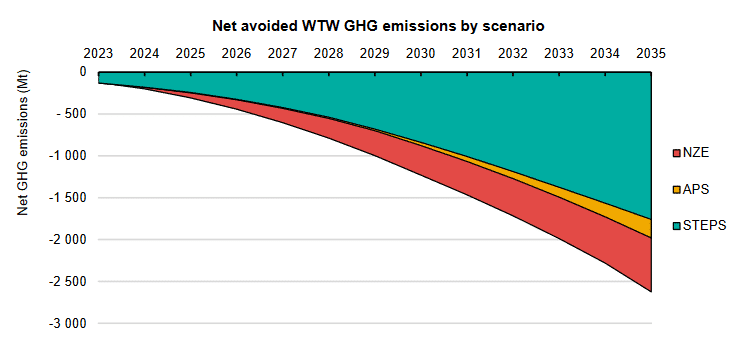
Lithium mining and manufacturing is costly, but so is BURNING FOSSIL FUELS
Some EV opponents aim their criticism at the vehicles’ lithium-ion batteries, often claiming that manufacturing these batteries is environmentally unfriendly. While lithium-ion batteries are emissions-intensive to make, they replace the even more emissions-intensive diesel or gasoline that powers an ICEV. This replacement reduces overall emissions.
Lithium-ion batteries come in various shapes and sizes, but they’re all built on a common design: a lithium-based cathode at one end; a carbon- or metal-based anode at the other end; and a solvent in between, filled with the titular lithium ions. As an EV is driven around, the lithium ions flow from the anode to the cathode, draining the battery of charge. When the EV is plugged into a charger, the ions flow in reverse and pile up back at the anode.

Often, the cathodes are the most emissions-intensive parts of the battery to make6. Usually, the cathode is made from an oxide of lithium mixed with a medley of other metals. After gathering the raw materials (which are often emissions-intensive to extract), they must be prepared into a battery-ready form, a step that often requires heating the mixture to around 800°C: a temperature that industry can only reach cost-effectively by directly burning coal or natural gas. Finally, the finished cathodes and other parts must be packed into a battery. Most EV makers use batteries with cathodes made from lithium, nickel, manganese, and cobalt (also known as NMC), but Tesla and some other manufacturers now use batteries with lithium iron phosphate (LFP) cathodes, which are responsible for lower overall emissions overall (about 30% lower emissions per kilowatt-hour, according to the IEA)1.
Manufacturing a battery involves a good deal of electricity, and a battery’s emissions will obviously increase if higher-carbon-intensity electricity is used to manufacture it. This is especially relevant when more than two-thirds of the world’s lithium-ion batteries are manufactured in China, where coal is the primary energy source for both electricity and industrial heating. Indeed, building a lithium-ion battery in some parts of China can account for more than twice as many greenhouse gas as building an identical battery in Scandinavia, France, or California7.
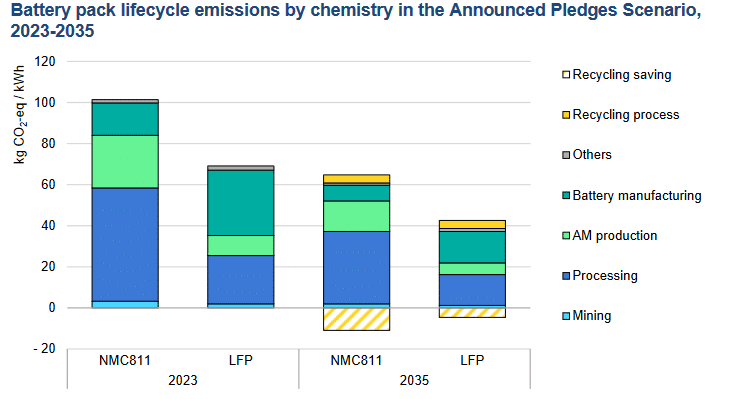
EV critics often cherry-pick some of the above details to portray EVs as sneakily carbon-intensive. When they do so, they ignore crucial context: that ICEVs generate far more emissions over their lifetime, as we’ve shown.
The amount of metal that goes into an EV’s battery is tiny compared to the amount of fuel that an ICEV uses. A single small vehicle’s lithium-ion battery pack might use no more than around 120 kg of metal, though this varies with a vehicle’s weight and battery size. On the other hand, according to a study by advocacy group Transport and Environment, the average ICE-powered car will burn through either about 12,600 kg of petrol or about 11,400 kg of diesel over the course of its lifetime.
This is because, while the metals only enter an EV during manufacturing (or if its battery gets replaced), an ICEV will continue to use fuel and rack up tank-to-wheel emissions for as long as it operates. As an EV avoids those emissions, it can make up for its higher production emissions on the road.
Imagine driving two vehicles: an EV and its ICEV counterpart. The EV may start its first drive on the road with a larger footprint than the ICEV – a difference sometimes called a “carbon debt” – but it can quickly “pay off” that difference as both are driven. The difference will shrink, and after a certain distance driven, the ICEV’s footprint will surpass the EV’s. From then on, the difference between the EV and ICEV will continue to grow, in the EV’s favor.

How quickly an EV “pays off” its carbon debt depends on the type of vehicle and where it’s being driven. Logically, an EV will be more effective if it is charged on electricity from cleaner, lower-carbon sources. As an example, take a Nissan Leaf and compare its emissions to those of a Fiat 500, an equivalent ICEV. Assuming each car is driven for 14,000 miles (22,400 km) per year, the average distance in the US, and assuming the Nissan Leaf is charged on the average US electricity mix, the Nissan Leaf will reduce its emissions beneath the Fiat 500 by the end of its second year on the road. The period will be even shorter if the electricity comes from a lower-carbon source. In comparison, the average US vehicle is 12.2 years old as of July 2023. If both the Fiat 500 and the Nissan Leaf last that long, the Leaf will be responsible for less than half the emissions of the Fiat 500 (see Figure 3).
Both industrial metals and oil do, of course, come with other environmental costs. However, we will discuss those impacts in a later article. In this one, we focus on the CO2 emissions and show that, in most cases, a lithium-ion-battery powered EV is responsible for less emissions than an equivalent ICEV.
An EV has an emissions advantage in most countries
Detractors often claim that, because an EV pushes emissions away from the vehicle and to the power plants that generate its electricity, it is not cleaner than a comparable ICEV. Plentiful evidence shows that this claim is almost always false.
EVs can run on electricity generated by low-carbon sources like renewables and nuclear power. The Intergovernmental Panel on Climate Change (IPCC) collated data on the lifetime carbon footprint per passenger per distance driven for a number of different vehicles and energy sources. Their data indicates that, when an EV runs on low-carbon electricity, it produces the absolute lowest emissions of any type of vehicle in the dataset – including ICEVs running on fossil fuels, ICEVs running on biofuels, hybrid vehicles, and hydrogen fuel cell vehicles2.
Of course, that scenario belongs to an ideal, zero-carbon world. What about one closer to today’s world, where most electric grids still have fossil fuels in their mix? The IPCC’s data suggests that an EV has an advantage on average over a gasoline- or diesel-powered vehicle even when the EV runs on entirely natural-gas-powered electricity. Only when an EV runs on electricity generated entirely from coal – the most carbon-intensive fossil fuel – do its emissions compare to those of a combustion vehicle2. But virtually nowhere in the world has a fully coal-dependent electrical grid.
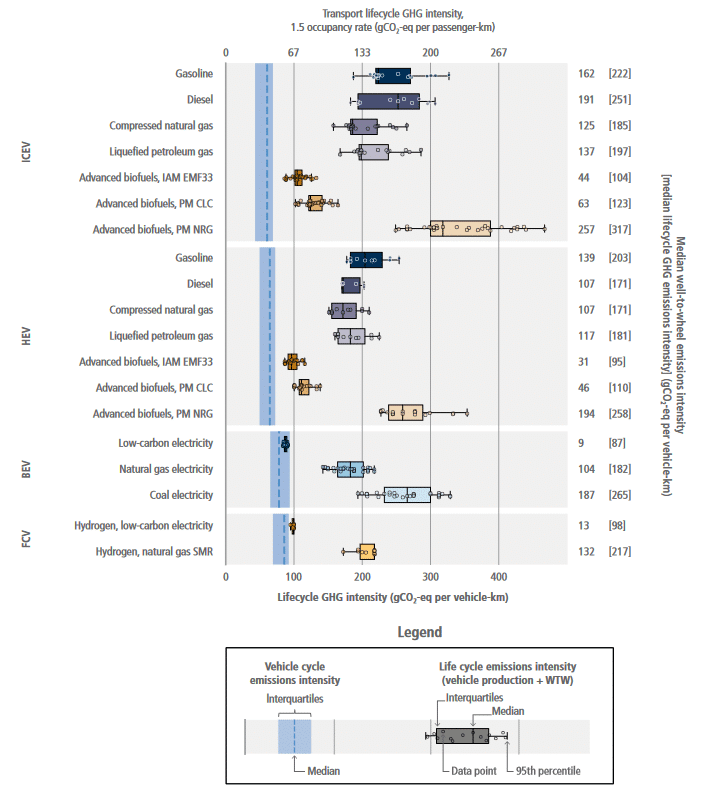
The IPCC’s data looked at hypothetical abstract scenarios, not real locations. However, several other studies have pitted EVs against ICEVs on a real-world, country-by-country basis and found similar results – that EVs usually have the emissions advantage. For example, one study conducted by Knobloch and colleagues analyzed 2015 data. Its authors divided the world into 59 countries and regions. In 53 of those regions, together accounting for 95% of the globe’s transport demand, an EV reduced the ICEV’s lifetime emissions on average3.
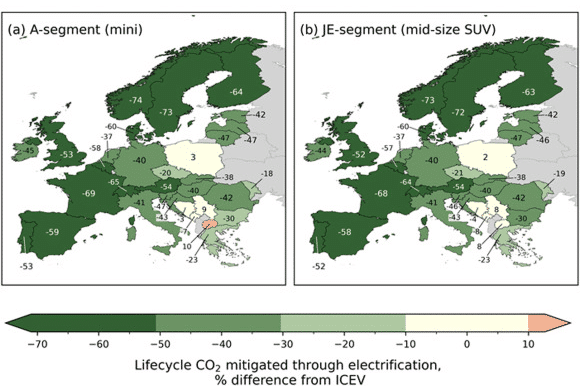
Other studies agree with this conclusion. A second study compared mini and mid-sized EVs with ICEVs across 32 European countries; in 29 of them, the EVs were responsible for fewer lifecycle emissions on average than their combustion-powered counterparts, as shown in Figure 54. Another study, conducted by Sacchi and collaborators, compared EVs with hybrid electric vehicles across 35 countries. Even though gasoline-powered hybrids have lower emissions than conventional ICEVs, EVs still had the emissions advantage over hybrids in 26 of the 35 countries5.
The EV advantage will grow in the future
We’ve seen that, today, EVs have a clear lifetime advantage over ICEVs. But we can also see that an EV’s emissions advantage is predicted to grow.
For example, if the world continues to decarbonize its electrical grids, an EV’s well-to-tank emissions will decrease in tandem. We cannot stop CO2 from being emitted when we burn fossil fuels. We can, however, reduce the amount of CO2 required to produce a certain amount of electricity: namely, by reducing the amount of fossil fuels in the grid mix. The IEA predicts that, in 2035, an EV’s lifecycle emissions will be 60% lower than an equivalent ICEV on average in a scenario matching current policies and 70% lower in a scenario corresponding to countries’ announced pledges1.
Knobloch and colleagues, who compared the lifecycle emissions of equivalent ICEVs and EVs in 59 different divisions, also projected emissions in 2035 and 2050 under two scenarios: one corresponding to current trajectories and a second that limits global warming to 2°C by century’s end. The study found that, in almost every part of the world, the EV’s advantage over a comparable ICEV deepens in both scenarios3.

Knobloch told Science Feedback the following in a previous insight:
Since 2015, renewable electricity generation has grown considerably all around the world – much faster than what we had modeled in our scenarios. As a result, the climate benefit of electric cars is also growing much faster than expected. Petrol-powered cars, on the other hand, can hardly become more efficient. This makes the electrification of cars an absolute no-brainer.
Sacchi and collaborators also estimated EV and hybrid emissions out to 2050. Their study projected that, by then, an EV would be responsible for significantly lower emissions than the hybrid in 34 of the 35 countries in the study – the lone exception being India5.
There are clear ways to reduce an EV’s production emissions, too. As we have shown, making batteries in countries with lower-carbon energy mixes can reduce their footprint. Moreover, production emissions can be reduced by adopting more efficient manufacturing methods or turning to new battery types.
Recycling is another way to reduce emissions. The typical battery on the road today will likely outlast its car’s average lifetime, making either the battery’s materials or the whole battery ideal for reuse. Using recycled materials for new batteries can reduce their production emissions. Battery recycling is already happening, and the EU has set recycling targets: recovering 80% of lithium and 95% of other battery metals by 2032, then making new batteries with at least 12% recycled lithium, 15% recycled nickel, and 26% recycled cobalt by 2036.
According to a report prepared for the UK government, “The net result […] is that manufacturing related emissions are close to parity between BEVs and ICEs by 2050”8.
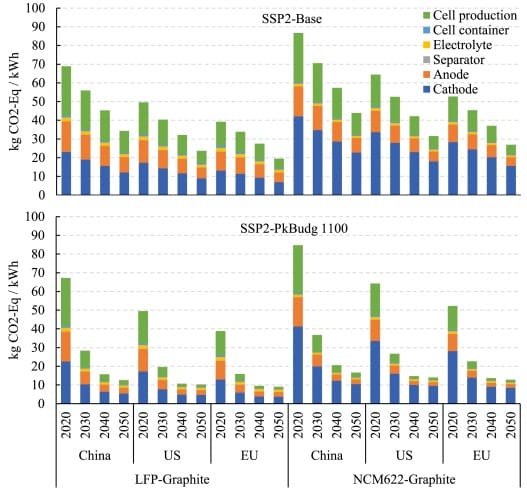
Claims that EVs are dirty because of their electrical sources often ignore these possibilities. But can ICEVs also become more efficient? The answer seems to be yes, to a degree. According to analysts from Argonne National Laboratory, future innovations could decrease the lifetime emissions of a gasoline-powered mid-sized car by 25% and those of a diesel-powered equivalent by 18% – compared to between 27 and 32% reductions for battery-powered mid-sized cars (which start with lower lifetime emissions today)10.
But the same analysis estimated that a complete decarbonization of the electric grid would reduce EV emissions by 73 to 77%, compared to the present day. Meanwhile, gasoline- or diesel-powered vehicles will not see those kinds of drastic emissions cuts without swapping out their petrol or diesel for biofuels, synthetic fuels, or other alternatives. Switching to an entirely renewable energy grid is, at this moment, a more likely scenario than switching to those kinds of alternative fuels.
Conclusion
Ultimately, when people and governments talk about EVs, most do so because they want to reduce the greenhouse gas emissions related to road transport. So, when we talk about a vehicle’s emissions, it is important to look at all the emissions associated with that vehicle, not just during one stage of its lifetime. When we do so, we find that the EV usually has an advantage over an equivalent ICEV. Even if the EV starts its life on the road with higher emissions, it usually makes up for the difference simply by not burning fossil fuels in the vehicle.
Furthermore, evidence shows that EVs do not simply push their emissions onto the power plant. Multiple studies indicate that EV reduces an ICEV’s emissions in most scenarios, on all but the most fossil-fuel-dependent and highest-emissions electric grids. Studies have also predicted that an EV’s advantages are likely to grow in the future, through pathways that ICEVs likely cannot follow.
Scientists’ Feedback
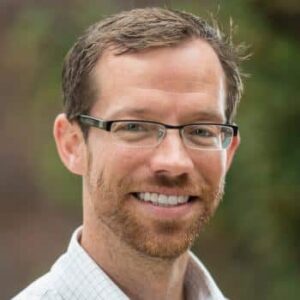
Jarod Kelly
Principal Energy Systems Analyst, Argonne National Laboratory
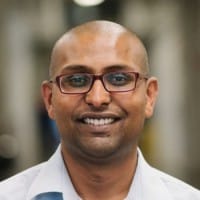
Rakesh Iyer
Energy Systems Analyst, Argonne National Laboratory
SF: What is the scientific consensus on how an EV compares to a combustion vehicle?
JK & RI: From the perspective of national and global decarbonization, there is clear scientific consensus that replacing internal combustion engine vehicles with electric vehicles reduces carbon emissions from a life-cycle perspective (i.e., accounting for vehicle emissions during vehicle production, well-to-pump, and pump-to-wheel emissions, and decommissioning). There are, however, other environmental concerns related to the use of electric vehicles, such as those related to toxicity, which may not yet have consensus.
SF: What aspects of an EV contribute the most to its life-cycle-wide CO2 emissions or footprint?
JK & RI: If we only talk about carbon (or greenhouse gas/GHG) emissions for electric vehicles (EVs), the biggest contributor to the EV’s life cycle carbon footprint is from carbon impacts associated with the electricity used for vehicle charging (because this electricity comes from fossil fuels like coal and natural gas). The next biggest contributor is the carbon impact contributions from (a) production of battery cathodes and anodes and (b) energy consumed for battery manufacturing.
SF: Can the footprint of an EV be reduced?
JK & RI: Again, if we focus on the life cycle carbon footprint of EVs, the most important measure to reduce this footprint is to use electricity generated using low-carbon energy sources (nuclear and renewables) for electricity used for EV charging and for the production of both battery constituents (like cathodes) and the final battery (battery assembly). Other measures would focus on replacing any fossil fuels (such as natural gas) with electricity or low-carbon energy sources for battery constituent production and battery assembly.
References
- 1 – International Energy Agency. (2024) Global EV Outlook 2024.
- 2 – Intergovernmental Panel on Climate Change. (2022) Transport. In: Climate Change 2022: Mitigation of Climate Change.
- 3 – Knobloch et al. (2020) Net emission reductions from electric cars and heat pumps in 59 world regions over time. Nature Sustainability.
- 4 – Hung et al. (2021) Regionalized climate footprints of battery electric vehicles in Europe. Journal of Cleaner Production.
- 5 – Sacchi et al. (2022) When, where and how can the electrification of passenger cars reduce greenhouse gas emissions? Renewable and Sustainable Energy Reviews.
- 6 – IVL Swedish Environmental Research Institute. (2019) Lithium-Ion Vehicle Battery Production.
- 7 – Kallistis et al. (2024) Think global act local: The dependency of global lithium-ion battery emissions on production location and material sources. Journal of Cleaner Production.
- 8 – UK Department for Transport. (2021) Lifecycle Analysis of UK Road Vehicles.
- 9 – Xu et al. (2022) Future greenhouse gas emissions of automotive lithium-ion battery cell production. Resources, Conservation and Recycling.
- 10 – Argonne National Laboratory. (2023) Cradle-to-grave lifecycle analysis of U.S. light-duty vehicle-fuel pathways: a greenhouse gas emissions and economic assessment of current (2020) and future (2030-2035) technologies.