- Energy
Environmental impacts of the fossil fuel industry outweigh those of EV metal mining, experts say
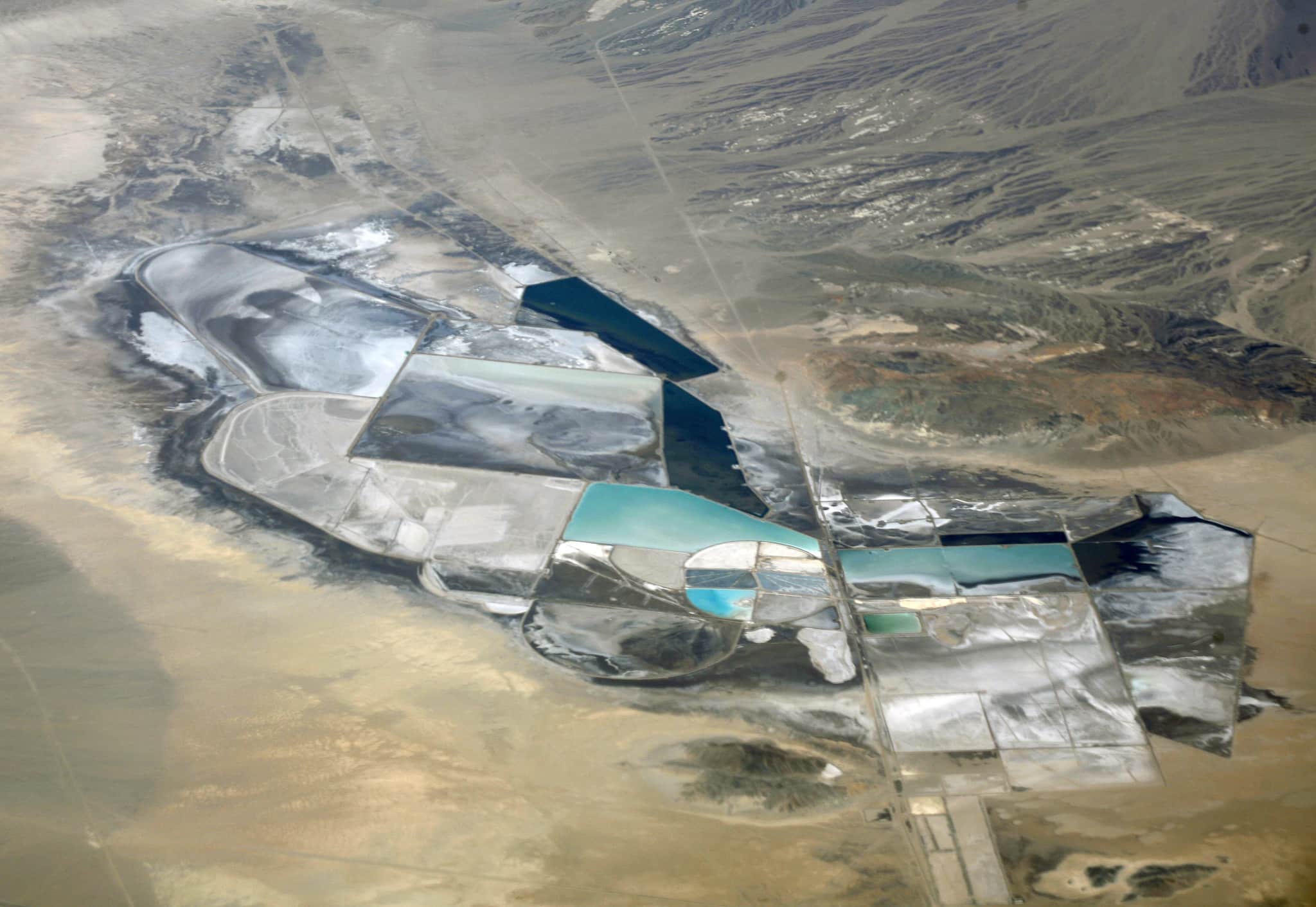
Nearly one in five new vehicles sold around the world in 2023 was an electric vehicle (EV), up from around one in seven sold in 20221. Swapping out gasoline- or diesel-fuelled combustion vehicles in favor of EVs is an unequivocally good way to reduce greenhouse gas emissions from transportation – as Science Feedback has covered, EVs emit less CO2 over the course of their lifetime in all but the most fossil-fuel-dependent electric grids.
Instead of gasoline or diesel, EVs run on a lithium-ion battery, which contains lithium as well as other metals such as cobalt and nickel. Mining these metals is not free of consequence. As Wojciech Mrozik, Senior Research Fellow at Newcastle University, summarized to Science Feedback:
“There are several critical issues throughout the battery lifecycle, particularly related to the extraction of key materials like cobalt, nickel, and lithium. Cobalt, however, is mostly a by-product of copper mining, and is associated with child labour; nickel mining causes extensive water pollution (evident in satellite images of mines in Norilsk, Russia); and lithium extraction from brine depletes and contaminates water in regions already facing scarcity, such as South American deserts.”
Since most combustion vehicles lack lithium-ion batteries, it’s often claimed that EVs’ metal needs make EVs less environmentally friendly than combustion vehicles (for example, see this image that Science Feedback has previously reviewed). As we show in this insight, these claims are fundamentally misleading. We can’t directly compare the total environmental impacts of gasoline and diesel to those of battery metals – they’re different. But we can say that petroleum isn’t a clean energy source by any standard – extracting, refining, and burning it all produce pollutants, not to mention the greenhouse gasses.
We can also say that experts believe making batteries is necessary to reduce the even more disastrous consequences of climate change, caused in large part by those very greenhouse gasses. Metal mining does have unfortunate negative impacts, but researchers don’t believe they outweigh the need to decarbonize. In fact, there is evidence that a transition to a zero-carbon energy grid will actually reduce the total amount of energy-related mining, by reducing the world’s reliance on coal.
Main Takeaways:
- Both petroleum extraction for vehicle fuel and metal mining for batteries pollute their environment. The nature of the pollution is different between the two sources, however, meaning they can’t easily be compared to one another.
- However, we can say that petroleum extraction is not a clean energy source by any means. Every step of the supply chain that brings fuel to a vehicle spawns pollutants and inflicts documented harm to the environment and human health.
- Electrifying vehicles reduces greenhouse gas emissions and the even more destructive effects of greenhouse-gas-induced climate change. In that context, scientists don’t believe that the pollution from battery metal mining is sufficient reason to avoid the transition to electric vehicles. In fact, a transition to net-zero emissions could reduce the total amount of energy-related mining by loosening the world’s reliance on coal.
- Researchers are well aware of battery metal mining’s impacts. There is a good deal of work being done on ways to reduce those impacts, such as battery recycling or new battery chemistries.
Petroleum is not a clean energy source
First, gasoline and diesel are not “clean” or “green” by any standard. Most obviously, burning them as fuel produces CO2 and other greenhouse gasses. As Science Feedback has shown before, and as is the overwhelming consensus amongst climate scientists, greenhouse gasses are the primary cause of observed climate change.
Beyond greenhouse gas emissions, extracting fossil fuels and burning them for energy (whether to generate electricity, to provide heat, or to power vehicles) comes with steep environmental costs. It’s easy to gloss over this burden or take it for granted. But every step of bringing fossil fuels to the car engine – including extracting crude oil, refining it into usable fuel, burning that fuel in the engine, and transporting all of it from place to place – does well-documented damage to both human health and the environment.
Most crude oil is extracted by drilling a borehole into the ground or under the sea floor and pumping crude oil up and out through the shaft. It’s well-known that drill sites generate pollutants like sulfur dioxide and hydrogen sulfide and carcinogens like the so-called BTEX compounds: benzene, toluene, ethylbenzene and xylene2. Even after an oil well closes, it can leak hydrogen sulfide, benzene, arsenic, and methane. These pollutants enter air, soil, and groundwater, including human drinking water.
Oil wells also create waste – a mixture of shredded rocks from the borehole and industrial fluids poured into the well to boost production – that’s rich in toxic heavy metals and polycyclic aromatic hydrocarbons (PAHs), pollutants that can cause cancer and genetic damage in humans3. Different studies, conducted across multiple continents, have linked these pollutants to increased risks of cancer, respiratory illnesses, and skin diseases for oil workers and non-workers alike. There’s also evidence that drill waste degrades the quality of local water by increasing its salinity and chloride levels2.
Crude oil then comes to the refinery. Crude oil is a blend of many different hydrocarbon molecules, and a refinery uses a cluster of chemical processes to sort and crack the hydrocarbons, separating crude oil into substances like asphalt, motor oil, kerosene, liquid petroleum gas, and, indeed, gasoline and diesel. The U.S. Environmental Protection Agency (EPA) labels petroleum refineries as major sources of countless air pollutants: airborne BTEX compounds, hydrogen sulfide, sulfur dioxide, carbon monoxide, nitrogen oxides, and particulate matter, to name a few. Numerous studies have found that residents living near petroleum refineries suffer increased rates of cancer (especially blood cancer) and respiratory illnesses4,5.
Not only does producing gasoline and diesel pollute the air, but so does burning it. In addition to greenhouse gasses, combustion vehicle tailpipes spew out fine particulates and ozone. These pollutants spread around the world and aggravate respiratory diseases. One study estimated that the particulates and ozone from gasoline vehicles caused between 139,700 and 170,700 premature deaths around the world every year between 2000 and 2015 on average; the study attributed between 205,000 and 309,300 annual deaths to the same pollutants from diesel6. (These numbers may be small fractions of the total number of deaths attributed to burning all fossil fuels, estimated at around 5 million each year.)
Crude oil inadvertently spills into its surroundings at every step of the way. Drill sites leak and leach oil and fluids into their surroundings, contaminating soil and water alike2. Spills can happen while transporting the crude oil from the drill site to the refinery, while transporting fuel from the refinery to the vehicle, and even if a fuel tank leaks in storage. Oil can even trickle into the environment when vehicles leak or fuel or motor oil onto pavement and rain carries it away – such runoff is actually the number one source of oil pollution in North American oceans.
When you imagine an oil spill, you might picture headline-grabbers like Deepwater Horizon (an offshore rig that suffered a catastrophic failure and spewed some 172 million gallons of crude oil into the Gulf of Mexico) or oil tanker accidents like those of the Exxon Valdez and the MT Sanchi. Large spills like these have become less common since the 1970s thanks to better infrastructure, but they’re only a small fraction of the hundreds of millions of gallons of oil spilled every year. 80% of those tanker spills are smaller than 7 tonnes (about 2,100 gallons) at a time7. According to a mid-2000s estimate by the U.S. EPA, 2.5 billion gallons of petroleum were spilled in the U.S. each year alone8.
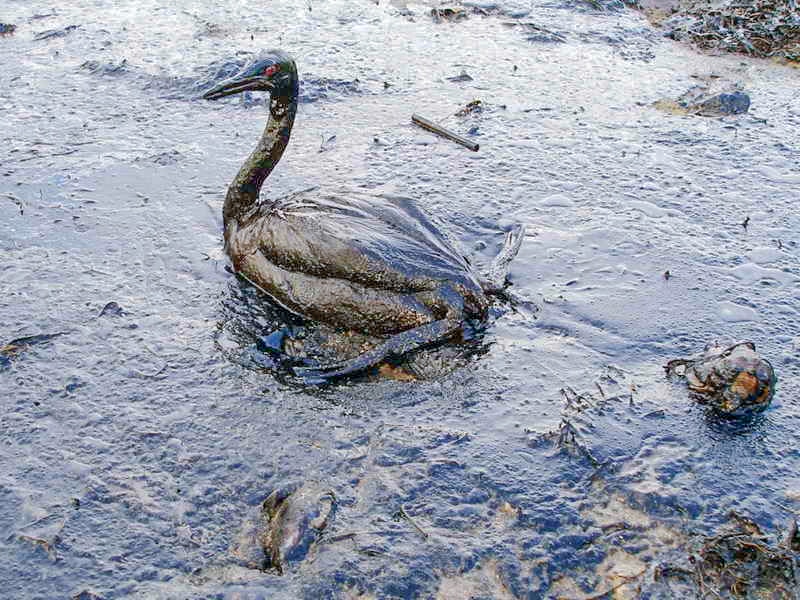
Petroleum forms naturally, mostly when the remains of living things – hence the name “fossil fuel” – are buried by stagnant water, dirt, or rocks. Under the right conditions, instead of decomposing as they would on the surface, their remains break down over millions of years into pools of hydrocarbons: crude oil or natural gas. But a natural substance isn’t necessarily a non-toxic substance, and petroleum’s natural origin certainly doesn’t stop its negative impacts. Thick, viscous crude oil can literally smother wildlife (see Figure 1). If petroleum or its products enter soil and water, even in small amounts, they can leave behind heavy metals (including arsenic), PAHs, and BTEX compounds, which as we’ve mentioned are toxic to both humans and wildlife who live on the soil or drink the water2.
Certain battery metals have environmental consequences
We’ve established that battery-using EVs are good for decarbonization, but we’ve also established that batteries contain metals that are not consequence-free to mine. EV critics often portray these consequences as “hidden costs”, but the scientific community is well aware that battery metals have environmental impacts. So, what are those impacts?
Lithium-ion batteries come in all sorts of shapes and sizes, but they all share a common basic design: a negatively charged anode on one end (usually made from graphite) and a positively charged cathode on the other (usually fashioned from a blend of lithium and other metals), sandwiching a solvent filled with lithium ions (which give the battery its name). When an EV is driven around, its battery discharges, and the ions flow through the solvent to the cathode. When an EV is plugged in to charge back up, the ions flow back to the anode.
Most lithium today comes from one of two sources: hard-rock ores and continental brines. Extracting lithium hard-rock ore is like traditional mining: extractors dig for lithium-rich minerals, such as spodumene, which are then processed to extract the lithium. Continental brines, on the other hand, are subterranean reservoirs of salty water rich in dissolved lithium; the water is pumped into large pools on the surface and left to slowly evaporate over months, leaving lithium salt behind (see Figure 2). As of 2018, brines provided about two-thirds of the world’s lithium9.
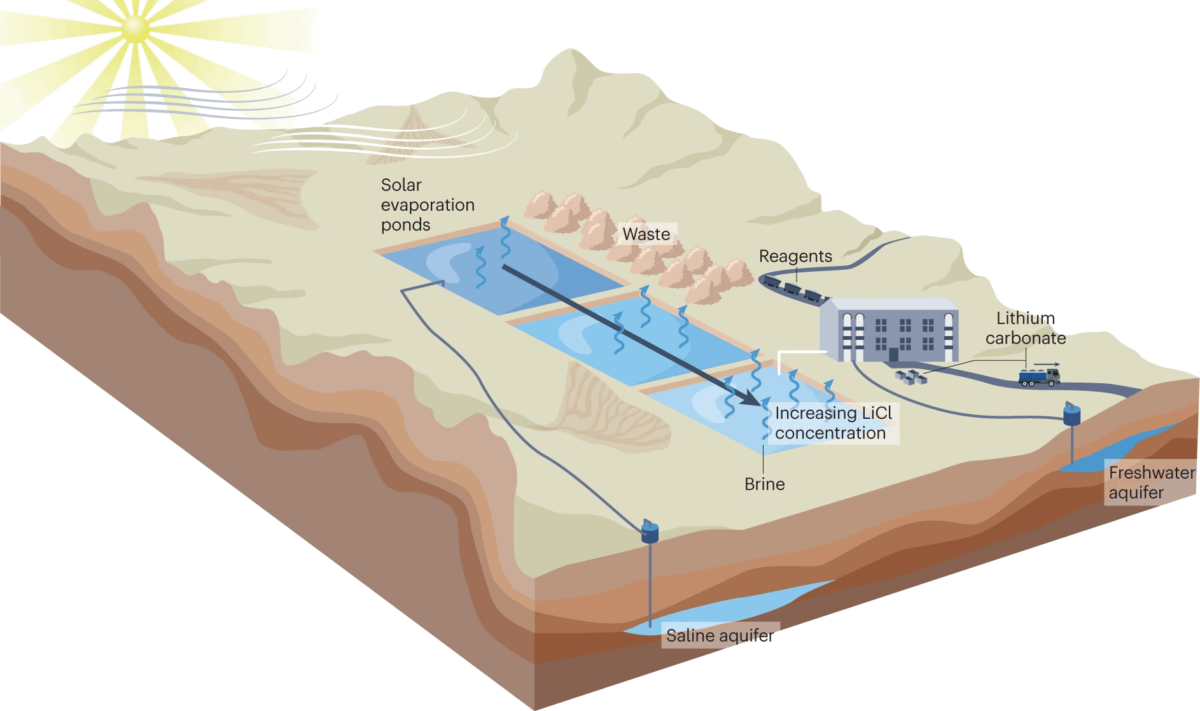
Hard-rock lithium casts a larger CO2 footprint than brine lithium, and sifting lithium from the ore is usually done with acid, a chemical process that’s known to spawn sulfur-based pollutants11. On the other hand, while the more widely used brine lithium produces less pollution, it isn’t waste- or consequence-free. In particular, brine lithium has garnered criticism for its water use. As much as 90% of the brine water evaporates into the atmosphere10. The evaporating brine may be salty water, but fresh water is often used to extract the finished lithium carbonate from the brine. This water consumption may deplete water from and desertify a lithium mine’s surroundings; there’s evidence that flamingo populations in northern Chile, one of the world’s lithium brine hotspots, have dropped following reduced water levels, especially in winter10.
A lithium-ion battery’s cathode contains most of its lithium, but as mentioned, lithium isn’t the only material in a cathode. There are a few different cathode types, differentiated by the addition of different metals. Most EVs use lithium nickel manganese cobalt oxide cathodes (NMC cathodes, for short). Aside from lithium, reviewers highlighted to Science Feedback that NMC cathodes contain two problematic metals: nickel and cobalt.
Nickel comes in two types of ores: laterites (usually found in the tropics) and sulfides (usually found elsewhere in the world). In both cases, the nickel must be painstakingly separated from the ore, often done by treating the ore with acid. The separation process creates heavy-metal-containing waste, which has been recorded to enter nearby water and lead to die-offs of marine life. Processing sulfide ores in particular tends to pollute the air with sulfur dioxide. A microcosm of these effects is the Russian Arctic city of Norilsk (see Figure 3).
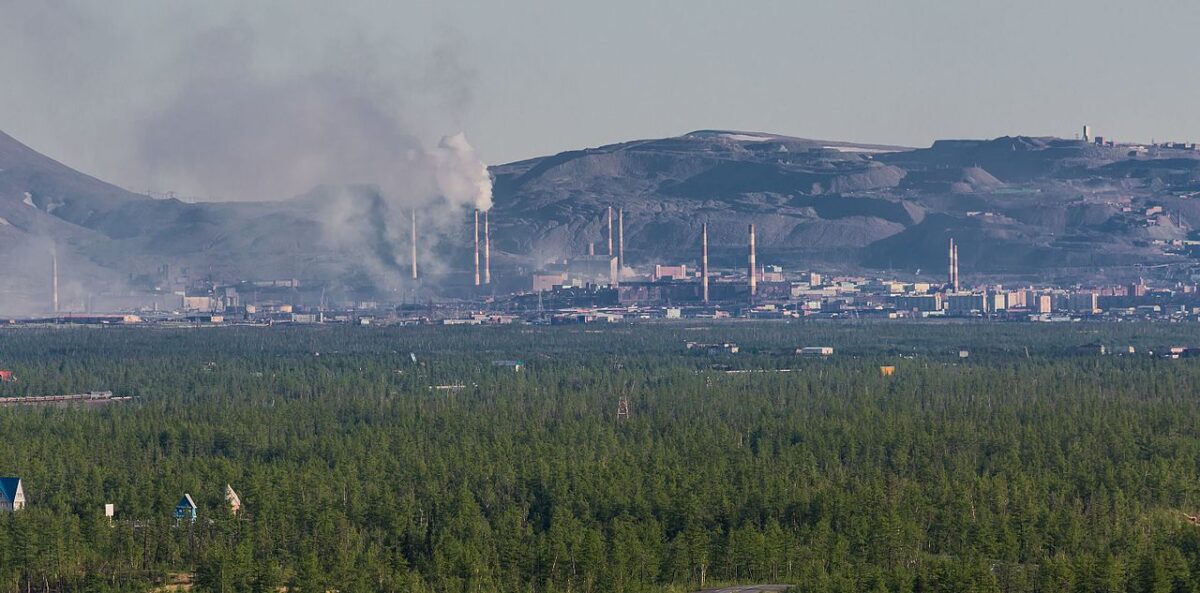
Determining cobalt’s environmental impact is more complex, because cobalt is rarely mined by itself. Instead, most cobalt is a byproduct of mining copper (or, more rarely, nickel). That said, we do know that separating cobalt from the ore also produces toxic waste, which tends to enter the water and kill marine life. Compounding the waste problem is that about three-fourths of the world’s cobalt comes from the Democratic Republic of the Congo, where many workers – often children or forced laborers – dig for metal ore with improvised equipment and lax safety measures due to poor regulation. These workers might be forced to breathe in toxic cobalt dust, aggravating respiratory issues and skin conditions. Residents living near Congolese cobalt mines have widely reported decreased crop yields, skin problems, and increased reproductive health issues. One study in the southern Congolese city of Lubumbashi linked exposure to copper and cobalt mining byproducts to birth defects11.
A transition to net-zero may reduce the overall amount of energy-related mining
We’ve also seen that certain metals used in EV batteries have negative environmental impacts. We’ve also shown that, even leaving aside the CO2 emissions from vehicle tailpipes, petroleum is a polluting energy source by any standard. It’s not possible to directly compare the two – the environmental impacts of battery metal mining are quite different from those of oil extraction, both in scope and in the type of impacts that we observe.
But scientists believe that the consequences of battery production do not outweigh the more disastrous consequences of fossil-fuel-driven climate change. The recorded impacts of climate change are widespread, ranging from distressed ecosystems to increased water shortages to rising risks for natural disasters, and affect every part of the globe.
Tim Werner, Senior Lecturer in Energy Transition at the University of Melbourne, commented to Science Feedback:
“We need to tackle climate change urgently, and an energy transition away from fossil fuels is key to this. We have no viable alternative on this front. An energy transition requires that we mine minerals. So, the key challenge ahead of us is not to decide whether or not to mine them, but rather where and how we can mine them with the lowest impact possible.”
If we keep making EVs with current technologies, we’ll need lithium, nickel, and cobalt in larger quantities. These metals also have other clean energy uses outside EVs: lithium, for example, will be needed if the world is to connect batteries to the electric grid on a large scale; wind turbines often use nickel in their moving parts. The IEA estimates that a net-zero scenario would require six times more metal mining in 2040 than in 202112.
But these claims have to be placed into their proper context. Fossil fuels are also not limited to vehicles, and the world’s energy system is already dependent on mining. Oil may not be mined in the traditional sense, but fellow fossil fuel coal certainly is. In fact, the total mass of battery metals mined in 2021 was less than one-tenth the mass of coal mined in 2021 alone13.
Because transitioning to a net-zero energy system will reduce the world’s dependence on coal, some researchers believe that aggressively electrifying the vehicle fleet as part of that broader transition will actually reduce the global total of energy-related mining (Figure 4). One study suggested that, by drastically cutting coal mining, achieving net-zero could reduce the total mined mass of energy-related material by about 45% from present-day levels13. Moreover, another published perspective suggested that, over the course of two decades, mining the same quantity of coal and wind power raw materials will generate five times more wind power14.
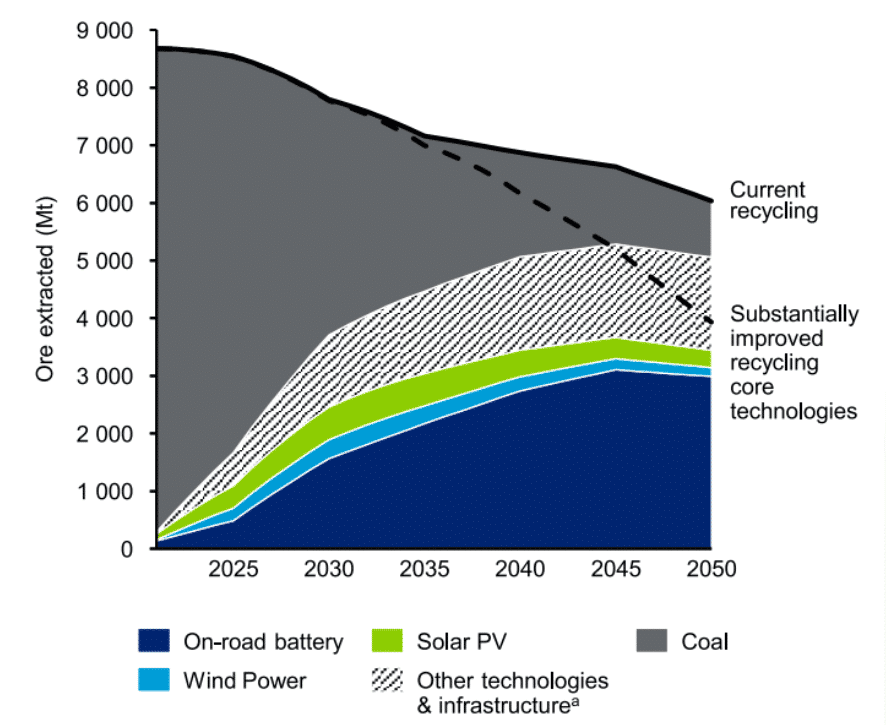
Werner, who co-authored one of the above-mentioned studies, commented to Science Feedback:
“This doesn’t mean that we should approve every single metals project. However, we do have an opportunity to be smart about which projects we approve, so that the energy transition is achieved at the lowest cost to people and the environment. This is why there is rightfully so much scrutiny on the impacts of battery projects. In summary, the [environmental] costs of mining battery minerals do not explicitly outweigh their benefits, and indeed they may help to accelerate the closure and rehabilitation of fossil fuel mine sites around the world.”
Some claims hold that electrifying road vehicles will simply push CO2 emissions from the tailpipe to the power plant, but evidence suggests that this is not true. According to data collated by the Intergovernmental Panel on Climate Change, if we examine the CO2 emissions from the entirety of a vehicle’s lifecycle, an EV emits fewer emissions per passenger per kilometer than a comparable combustion vehicle as long as the EV is powered by any type of electricity save for coal15. The IPCC’s report presented its data as a set of hypothetical scenarios, but as we show in our prior insight, studies comparing EVs and their combustion-powered counterparts around the world have found that their lifecycle emissions are comparable only in countries with the most coal-dependent power grids.
We can reduce EVs’ lithium, cobalt, and nickel consumption
It’s worth noting that a good deal of research is dedicated to cutting EVs’ (and, more broadly, the energy system’s) need for lithium, nickel, and cobalt.
One possibility is that we can reduce our need to mine these metals by recycling them, such as from used batteries. We can only recycle a small fraction of the world’s EV batteries today, but the world’s lithium-ion recycling capacity is expected to increase at least fourfold by 2030 (see Figure 5 )1. It helps that regulators are pushing for recycling. In 2023, the European Union enacted a law mandating that, by 2031, 6% of the lithium, 6% of the nickel, and 16% of the cobalt in new lithium-ion batteries must come from recycled sources – those targets rising to 12%, 15%, and 26%, respectively, by 2036. It’s estimated that, in a net-zero scenario, advanced recycling may cut the total mass of material mined for energy by about another third by 205012.
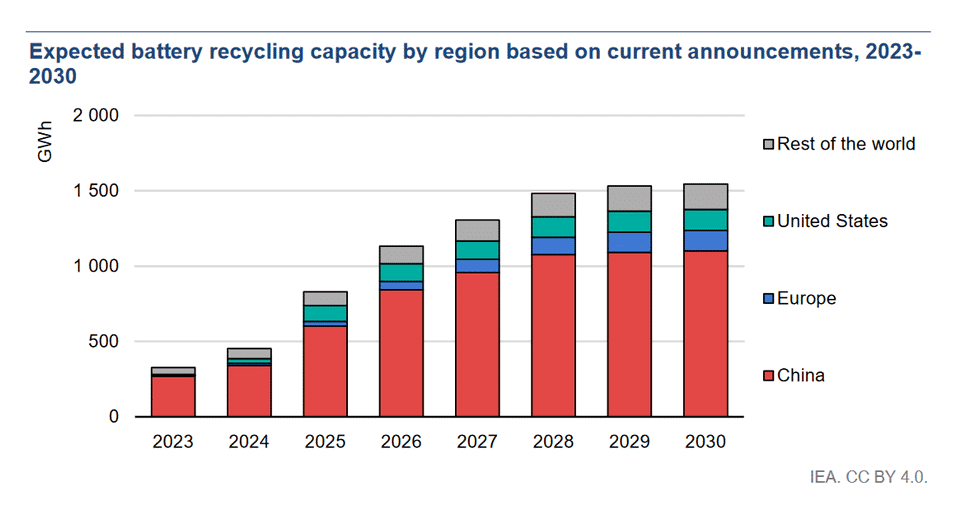
EV-makers can also reduce the amount of nickel and cobalt needed in lithium-ion batteries by choosing alternative cathodes that don’t contain either metal. The most popular alternative choice is the lithium iron phosphate (LFP) cathode. LFP cathodes are already common in China, where they power 70% of new EVs that hit the road in 20231. The rest of the world has been much slower to embrace LFP cathodes, but some EV manufacturers have done so: Tesla switched to LFP batteries in 2021.
More ambitiously, some researchers are trying to create rechargeable batteries that don’t rely on lithium at all. One popular idea is to swap out lithium for sodium, an element that is 1,000 times more abundant than lithium in Earth’s crust, making it less difficult and less ecologically damaging to attain. Sodium-ion batteries are still years, if not decades, away from use in road vehicles, but one preliminary study from 2021 stated that some sodium-ion batteries’ “environmental performance is getting close to or even better than that of their [lithium-ion battery] counterparts, and the remaining progress required for reaching similar values can be considered achievable, considering their still much lower technological maturity”16.
Conclusion
In short, experts do not believe that the impacts of battery metal mining are reasons to delay a transition from combustion-vehicle-powered vehicles to electric vehicles. On the contrary, experts say that batteries are necessary to combat climate change. At the same time, many researchers are well aware of the consequences from mining battery metals and are actively working to reduce the negative impacts of electrifying vehicles.
Moreover, it’s wildly inaccurate to claim that petroleum is any sort of clean energy source. Even brushing aside the greenhouse gas emissions from gasoline and diesel engines – which are partly to blame for driving recent climate change – every step of getting petroleum from an oil drilling site to the vehicle does harm to both human health and the environment.
Scientists’ Feedback
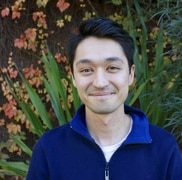
Tim T. Werner
Senior Lecturer in Energy Transition, University of Melbourne
SF: Are there particular social or environmental impacts from particular battery materials that you would want to highlight?
TW: The impacts of producing battery materials are diverse and depend heavily on which minerals are of interest, where they are produced from, and the circumstances of their production. Each mine and supply chain presents its own unique set of challenges that must be studied and managed. As such, there’s no particular issue worth highlighting over any other, because all are important.
I generally advise caution about over-emphasising the impacts of producing battery materials vs. the historical alternatives. Let’s not forget that the impacts of fossil fuel supply chains are also enormous. A recent study of mine into the world’s largest coal exporter (Indonesia), for example, shows massive social and environmental issues at play17.
SF: Are there particular battery materials that scientists or engineers are actively trying to reduce? If so, why, and how might their use be reduced?
TW: This is more of a question about supply security than one of social/environmental concern. Scientists and engineers are constantly developing new technologies that require different materials to different degrees. Sometimes when supplies of a material are cut short, efforts increase to develop alternative technologies that are less reliant on that material. At the same time, efforts might be increasing to find alternative sources, e.g. recycling, or mining from different countries. Countries and large companies often try to make strategic moves in response to perceived supply risks. For example, in a recent paper on rhenium, I mentioned two Jet engine manufacturers that were concerned about supply of rhenium18. One responded by redesigning their engines and supply chains so that they didn’t require as much rhenium. The other decided to acquire a mine.
Countries are also looking to diversify their supply chains and look into increasing recycling capacity. Recycling electric vehicles and electronic wastes might be a way to supplement some of our future needs, but we will continue to need a lot of mining in future.
I am not aware of one particular battery mineral whose social/environmental impacts stand out as being so egregious above all others that we are not considering to use them at all in future.
SF: Ultimately, do the costs of such materials give any reason to re-evaluate the transition to low-carbon energy?
TW: First and foremost, we need to tackle climate change urgently, and an energy transition away from fossil fuels is key to this. We have no viable alternative on this front. An energy transition requires that we mine minerals. So, the key challenge ahead of us is not to decide whether or not to mine them, but rather where and how we can mine them with the lowest impact possible.
Research has shown that overall, an energy transition would require significantly less mining13. So, quite aside from the benefits of climate change achieved, the impacts on landscapes would also be lower. This doesn’t mean that we should approve every single metals project. However, we do have an opportunity to be smart about which projects we approve, so that the energy transition is achieved at the lowest cost to people and the environment. This is why there is rightfully so much scrutiny on the impacts of battery projects.
In summary, the costs of mining battery minerals do not explicitly outweigh their benefits, and indeed they may help to accelerate the closure and rehabilitation of fossil fuel mine sites around the world.
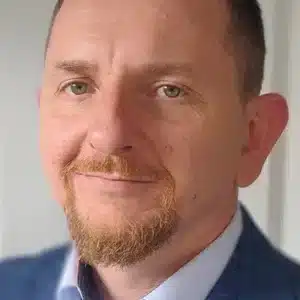
Wojciech Mrozik
Senior Research Fellow, Newcastle University
SF: Are there particular social or environmental impacts from particular battery materials that you would want to highlight?
WM: There are several critical issues throughout the battery lifecycle, particularly related to the extraction of key materials like cobalt, nickel, and lithium. Cobalt, however, is mostly a by-product of copper mining, and is associated with child labour; nickel mining causes extensive water pollution (evident in satellite images of mines in Norilsk, Russia); and lithium extraction from brine depletes and contaminates water in regions already facing scarcity, such as South American deserts.
During battery usage, failures can result in the release of harmful materials, including toxic and flammable gases, electrolytes, and nanometals. In the recycling and disposal phase, depending on the technology used, by-products like polluted water from hydrometallurgy or potentially toxic end-products like slag may be generated. If landfilled, batteries can interact with biomass gases, increasing fire risks, or releasing harmful substances, such as electrolytes, degradation products, gases, and nanometals, into the environment, potentially contaminating groundwater.
Recent studies have also indicated that batteries may be a significant source of PFAS (per- and polyfluorinated organic compounds).
Social impacts include living near mining sites or landfills, with illegal disposal and processing posing further risks (as in the case of E-waste). It’s observed in China, where is estimated that up to 70% of batteries intended for recycling are diverted elsewhere.
You can check more in my publication19.
SF: Are there particular battery materials that scientists or engineers are actively trying to reduce? If so, why, and how might their use be reduced?
WM: Yes, scientists and engineers are actively working to reduce the use of various battery materials, particularly lithium-ion batteries. Efforts include developing alternatives like niobium and solid-state batteries, replacing lithium with sodium, introducing new anode materials like silicon, and using water-based binders instead of PVDF. Another key focus is creating safer, less flammable electrolytes that are easier to recycle.
A prominent example is the reduction of cobalt, where new NMC chemistries use minimal cobalt, and cobalt-free LFP (lithium iron phosphate) batteries already surpassed the number of NMCs (e.g. in China it’s about 70% of the market)
SF: Ultimately, do the costs of such materials give any reason to re-evaluate the transition to low-carbon energy?
WM: None of the technology is free of environmental impact neither the oil industry nor battery. However, most of the needed elements for the batteries would be still mined as they are needed for other industries (even cobalt which is also a by-product of mining copper). Well, lithium is another story as we have developed more mines and brine processing just for the battery market.
I think the mistake was made that batteries were “sold” as a completely environmentally friendly solution without saying that they still need raw materials, thus mining. However, the stories about child labour (and it is mostly associated with artisan mines) prompted battery manufacturers to look for “ethical cobalt” and deal with the problem.
References
- International Energy Agency. (2024) Global EV Outlook 2024.
- Johnston et al. (2018) Impact of upstream oil extraction and environmental public health: a review of the evidence. Science of the Total Environment.
- Ilinykh et al. (2023) A life cycle assessment of drilling waste management: a case study of oil and gas condensate field in the north of western Siberia, Russia. Sustainable Environment Research.
- Domingo et al. (2020) Health risks for the population living near petrochemical industrial complexes. 1. Cancer risks: A review of the scientific literature. Environmental Research.
- Marquès et al. (2020) Health risks for the population living near petrochemical industrial complexes. 2. Adverse health outcomes other than cancer. Science of the Total Environment.
- Xiong et al. (2022) Long-term trends of impacts of global gasoline and diesel emissions on ambient PM2.5 and O3 pollution and the related health burden for 2000–2015. Environmental Research Letters.
- Brussard et al. (2016) Immediate ecotoxicological effects of short-lived oil spills on marine biota. Nature Communications.
- Azevedo C. (2007) Failure analysis of a crude oil pipeline. Engineering Failure Analysis.
- Flexer et al. (2018) Lithium recovery from brines: A vital raw material for green energies with a potential environmental impact in its mining and processing. Science of the Total Environment.
- Vera et al. (2023) Environmental impact of direct lithium extraction from brines. Nature Reviews Earth & Environment.
- Van Brusselen et al. (2020) Metal mining and birth defects: a case-control study in Lubumbashi, Democratic Republic of the Congo. The Lancet Planetary Health.
- International Energy Agency. (2022) The Role of Critical World Energy Outlook Special Report Minerals in Clean Energy Transitions.
- Nijnens et al. (2023) Energy transition will require substantially less mining than the current fossil system. Joule.
- Krane and Idel. (2021) More transitions, less risk: How renewable energy reduces risks from mining, trade and political dependence. Energy Research & Social Science.
- Intergovernmental Panel on Climate Change. (2022) Transport. In: Climate Change 2022: Mitigation of Climate Change.
- Peters et al. (2021) On the environmental competitiveness of sodium-ion batteries under a full life cycle perspective – a cell-chemistry specific modelling approach. Sustainable Energy & Fuels.
- Werner et al. (2023) Patterns of infringement, risk, and impact driven by coal mining permits in Indonesia. Ambio.
- Werner et al. (2023) Rhenium mineral resources: A global assessment. Resources Policy.
- Mrozik et al. (2021) Environmental impacts, pollution sources and pathways of spent lithium-ion batteries. Energy & Environmental Science.